Applications of MAIA the multispectral camera for environmental monitoring
The potential offered by RPAS in environmental awareness, prevention and monitoring is related to the possibility for sensors to fly over areas of interest. Remote Sensing in the environmental and territorial sector has undergone the first strong development with the NASA launch of the Landsat1 satellite in 1972, and since then numerous Earth Observation projects have been launched by numerous public and private space agencies.
In the last decade Proximity Remote Sensing technology saw its great development both with regard to sensors (lightweight multispectral and iperspectral sensors) and platforms (aircraft, balloons, helicopters, RPAS).
The platform is always equipped with a sensor, which can be active or passive. An active sensor emits electromagnetic radiation in the optical region, such as a LIDAR (Light Detection And Ranging) sensor, or SAR (Synthetic Aperture Radar): energy is reflected from the Earth’s surface and returns to the sensor where measurement is done. A passive sensor measures the physical and chemical data of the earth’s surface or the atmosphere on the basis of the reflected solar electromagnetic radiation or directly emitted by the objects in the investigated surface. A passive sensor can be optical or other type, such as those measuring meteorological parameters, air quality or ionizing or non-ionizing radiation. An optical sensor is characterized by the particular spectral region, within the entire electromagnetic spectrum, where the instrument works. The spectral region may include Visible (VIS), Near Infrared (NIR), Average Infrared (SWIR) and Thermal Infrared (TIR).

Figure 1 Electromagnetic spectrum in which the infrared regions are highlighted.
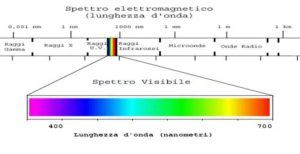
Figure 2 Electromagnetic spectrum in which the visible spectral region of the Visible is highlighted.
The number of its spectral bands characterizes an optical sensor: a panchromatic sensor operates in the visible region, a multispectral sensor provides images at different bandwidths and hence wavelengths, and a hyperspectral sensor is equipped with hundreds of very narrow bands.
The fundamental properties of the sensors are the geometric resolution, defined by the pixel size and therefore the ground information unit, the spectral resolution, i.e. the amplitude and variety of the bands, and the radiometric resolution, that is the sensitivity in the measurement that is able to return. The repetition rate of data acquisition finally defines the time resolution, which depends on the platform and not on the sensor.
MAIA WV is the multispectral camera with 9 sensors designed and developed with bands that have the same wavelength ranges as DigitalGlobe’s WorldView-2 satellite. It consists of an RGB sensor for real-life images, and 8 monochrome sensors with VIS-NIR spectrum sensitivity from 390 nm to 950 nm. Each sensor has a resolution of 1280×960 pixels (1.2 Megapixels) and the size of each sensor pixel is 3.75 μm x 3.75 μm. Monochrome sensors are coupled with band-pass filters that determine undesired wavelengths.

Figure 3 Electromagnetic Spectrum Detectable by MAIA WV-2 with relative wavelength intervals of the different spectral bands.
MAIA S2 is the 9-sensor multispectral chamber designed and developed to have 9 bands at the same wavelengths as ESA’s Sentinel-2 satellite. Each sensor has a resolution of 1280×960 pixels (1.2 Megapixels) and the size of each sensor pixel is 3.75 μm x 3.75 μm. Monochrome sensors are coupled with band-pass filters that determine undesired wavelengths.

Figure 4 Electromagnetic Spectrum Detectable by MAIA S2 with relative wavelength intervals of the different spectral bands.
Multispectral survey results are images unaffected by radial and geometric distortion, which present the pixel-pixel coregistration of information for all bands. Through the image processing software acquired with MAIA WV and MAIA S2, it is also possible to operate a radiometric correction of the multispectral data to obtain a repeatable and comparable data even under different light and environmental conditions.
For this reason, SAL Engineering and Eoptis have patented and developed ILS – Incident Light Sensor, an incident light sensor that records incident environmental radiation at every single shoot so that the multispectral data can be radiometrically corrected under the conditions of real and contingent lighting.
Different types of surface such as water, bare soil, or vegetation reflect radiation differently at the different wavelength ranges that define spectral bands: in this sense, the reflected radiation according to the wavelength is called spectral signature of the surface, which is proper and recognizable for certain elements and surfaces.
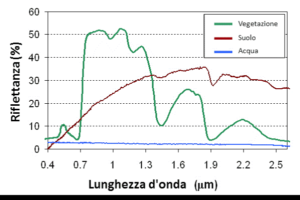
Figure 5 Spectral signature or spectral profile of vegetation, soil and water.
The vegetation has a very high reflection value in the near infrared and a low reflection value in the red channel of the visible: this allows for example to easily distinguish vegetation areas from those with bare soil through the RVI ( Ratio Vegetation Index), which is the ratio between quantified reflectance in NIR digital numbers and reflection in Red images.

Figure 6 Spectral sign of vegetation in the Visible and Near Infrared region.
It is possible to distinguish the dry vegetation from the wet vegetation, or to investigate the health of a crop by analyzing the curve of its spectral signature.
Dry vegetation does not absorb the red radiation typical of active photosynthesis, does not have the typical Red Edge reflection peak and does not exhibit the high reflection of NIR’s typical radiation incident.
The spectral signature of green plants is very characteristic: chlorophyll in a growing plant absorbs light in the visible, especially red, which it uses in photosynthesis. The near infrared light, on the contrary, is reflected very effectively because it does not serve the plant in any way: in this way the plants avoid excessive heating and evaporation of the lymph.
The vegetation reflection in the near infrared ranges and in the ranges of the visible varies considerably. The degree of difference reveals the extent of leafy vegetation in a portion of an area: in this sense a very important index is the Leaf Area Index (LAI), which is a very useful foliar index in agriculture and in management, for example, of a degraded area that has been regenerated or reclaimed.
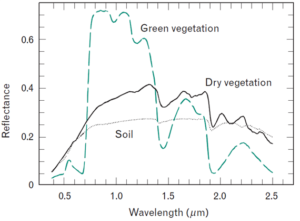
Figure 7 Spectral differences, recognizable in their spectral signature, dry vegetation and active photosynthesis vegetation.
The vegetation can be classified according to the specific spectral signature of the different plant species: in fact, research on quantitative biomass estimation and on the classification and monitoring of the tree species has already been carried out for decades thanks to multispectral surveys based on different spectral signatures of the tree species.
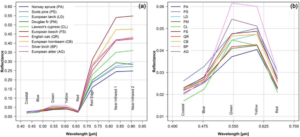
Figure 8 Different tree species classified according to their characteristic spectral signature.

Figure 9 Distinction of spectral reflection characteristics of conifers and hardwoods in the specific region of the Red Edge.
Each plant species and each agrarian culture is characterized by a specific phenological schedule. Multitemporal Remote Sensing allows you to observe phenological evolution during the year, and by comparing and predicting, to implement a plan for the prevention and monitoring of crops and the protection of natural forest, shelter, marsh or mountain ecosystems.
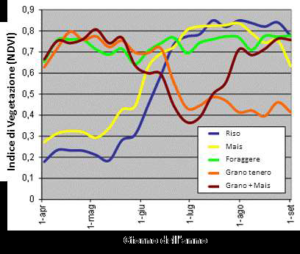
Figure 10 Distinctions in spectral reflectance characteristics between different crops.
There are also significant differences between different types of soil, in fact the multispectral survey is a large scale survey also used for the classification of geological soil: different mineral and lithological elements for their physical and chemical composition present a definite spectral signature. You can read a summary of applications of multispectral survey here. In addition, as with vegetation, it is possible to distinguish in terms of reflection a soil with high humidity from an arid soil because a soil with higher water content has a higher absorption of the incident and diffused radiation.
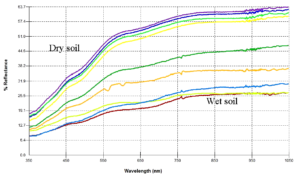
Figure 11 Distinction in spectral reflection between arid soil and wet soil.
Another very important matrix to be analyzed from a spectrometric point of view is water, whose variations in terms of spectral signature may characterize turbidity, the presence of suspended materials, or even contamination or the unexpected or unusual presence of suspended materials, or excessive or reduced production of phytoplankton in suspension. Generally water has minimal reflection only in the spectrum of the visible, and more precisely in the band of Blue and Violet. The reflection at these specified wavelength intervals allows a certain depth of penetration in the survey below the surface of the water bodies. With MAIA S2, equipped with a bandpass filter set that allows you to capture images at the same wavelengths of the ESA satellite Sentinel-2, and with MAIA in its WV filter set, that allow to capture multispectral data at the same wavelengths of the WorldView-2 satellite, it is possible to evaluate some water quality parameters:
- the concentration of suspended chlorophyll
- the presence of harmful algal blooms
- salinity and turbidity
- state of pollution or contamination of a water body.
It is also possible to distinguish within the flora in a water body, different species based on different reflection in the wavelengths of Blue and Violet. This knowledge is conducive to the safety assessment in all the exploitation activities that man will be able to implement of that water resource.
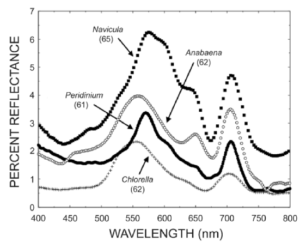
Figure 12 Comparison of spectra reflecting the taxonomies of four different algae in a water body, with almost identical chlorophyll concentration (written in parenthesis and expressed in μg / l).
In this report we also present high-quality scientific applications that the Research Institutes, Universities and Environmental Agencies have successfully tested, adding multispectral survey to well-established survey techniques, and in particular correlating information from precious multispectral data to the information already sought and documented in different fields of investigation of environmental science and knowledge of the territory.
The main measurements that can be obtained through a multispectral survey carried out with MAIA, concern:
Vegetation |
– Discrimination and classification of species |
|
– Estimation of biomass |
|
– Plant health status |
|
– Potential evapotranspiration |
|
– Real evapotranspiration |
Soil |
– Discrimination between different types of soil |
|
– Content of organic matter |
water |
– Content of turbidity |
|
– Concentration of chlorophyll |
Anthropized |
– Discrimination and classification of land use |
With regard to the sensors that SAL Engineering can make fly over the areas of interest, the main applications useful to an Environmental Protection Agency can be:
MAIA and MAIA S2
The Multispectral Camera |
· Classification of vegetation and health monitoring based on biophysical parameters; vegetation indices. |
|
· Identification and classification of land use, soil types, vegetation and crops with their health status.
· Analysis of the correlation between vegetation typologies and geomorphological aspects.
· Issues on agricultural production. |
|
· Evaluation of the environmental impact of the burned and then repopulated regions; tools for VIA (Environmental Impact Assessment) and VAS (Strategic Environmental Assessment). |
|
· Identification of unauthorized waste areas; monitoring of RSU dumps; identification of biogas emissions, location of percolate leakage and assessment of the health status of the surrounding vegetation. |
|
· Spill analysis found in water bodies; thermal behavior of surface water, mapping of algal types and their diffusion, torpidity and color of water, identification of paleoalve. |
|
· Digital 3D model of surface and ground; topographic profiles, level curves; orthophoto RGB and multispectral area of the area of interest. |
Thermal camera TIR |
· Thermal mapping of vegetation and crop anomalies. |
|
· Identification of areas with greatest fire risk; prediction areas of propagation; ongoing fire analysis. |
|
· Identification of spills of external material in water bodies; identification of floating or suspended material in water bodies. |
|
· Locating and mapping zones with thermal anomalies or differentials in landfill areas. |
|
· Creating georeferenced thermal video with database creation in GIS environment. |
High-Res Video camera |
· Creating video insights of phenomena and objects in inaccessible, dangerous areas. Creating geo-referenced videos with database creation in GIS environment. |
GAS Sensor |
· Measurement of the quantity of certain GASs for the determination of air quality. |
|
· Control the air quality during and after a fire in the areas adjacent to the event. Prediction of propagation areas during a fire. |
In the table above, applications of an aerial thermographic survey for environmental monitoring were reported, and we have previously reported the utility of correlating the multispectral data to a surface temperature evaluation of some objects and surfaces of interest. In fact, in the field of environmental protection, the applications of the RPAS thermal relief are numerous and in continuous exploration:
- It is possible to detect on the soil or in a forest any variation of temperature useful for the botanical or vegetative study of the species, and above all, to the prevention of fires and their propagation
- Water infiltration can be detected in landslides, rainwater can be monitored and remediation operations can be monitored.
- It is possible to detect thermal anomalies in free, woody or cultivated soils for the study of soil composition, to identify the coordinates of certain areas for possible geotechnical, geodynamic and geoelectric operations.
The RPAS thermographic survey has important application and development in the landfill areas, especially if the thermal orthophoto can be correlated with a multispectral orthophoto so as to associate to each pixel and thus to every small portion of the ground radiometric information thermal and multispectral.
The joint multispectral survey, as well as to identify and map the presence of hydrocarbons in the soil, serves to define and characterize the causes of the detected surface temperature differences.
In conclusion, by referring them to the reference environmental theme, below are the measurements and information that can be obtained by means of a survey conducted by SAL Engineering using MAIA optionally matched to a thermographic sensor:
Water quality |
Monitoring of eutrophic phenomena in water bodies such as mucilage, harmful algal blooms, chlorophyll content, suspended phytoplankton analysis or floating material analysis. |
|
Identification and identification of drains in water bodies. |
Soil quality |
Estimate organic content in the soil; identification and classification of minerals and metals in the soil. |
|
Identification and mapping of underground or natural anthropic structures. |
Hydrology, climate, agrometeorology |
Estimation of volumetric variations of glaciers; monitoring of frontal and periglacial areas. |
|
Classification of phenological status of crops. |
|
Mapping of nitrogen requirements in crops. |
|
Estimating the water needs of crops. |
|
Estimation of real and potential evapotranspiration. |
|
Monitoring soil moisture. |
|
Evaluation of damage from extreme atmospheric events, such as horns or air trumpets. |
Dams, lamination basins |
Measurement of sediment volume. |
|
Evaluation of the impact of the tax on water bodies downstream of the operations: assessment of torpidity, volumetric assessment of sediments. |
Conservation of ecosystems |
Monitoring of vegetation remediation. |
|
Monitoring of natural ecosystems. |
|
Monitoring and prevention of peat fires. |
|
Monitoring the phytosanitary and phenological status of natural vegetation. |
Construction sites |
Estimated volumes of lands and rocks moved. |
|
Monitor environmental impacts on natural vegetation and verify the correct restoration of the site at the end of the work. |
Air quality |
Measurement of air quality parameters near industrial plants and landfills. |
Geological instability |
Plano-altimetric survey of landslides, even on vertical walls. |
|
Monitoring of infiltrations and water circulation within rocky bodies; identification of cracks, faults. |
Avalanche |
Mapping areas of avalanche and estimating accumulation volumes. |
Quarries |
Estimates volumes captured at predefined timeframes. |
|
Verification of the correct restoration of the decommissioned quarries. |
Landfills |
Inspection of preliminary excavations; Insulation control and waterproofing and anti-infiltration. |
|
Checked volumes and volumetric estimates. |
|
Locating and mapping the percolation and multispectral analysis of the percussion physico-chemical composition. |
|
Identification and analysis of biogas emissions from RSU dumps. |
|
Identification of abusive landfills by analysis of alterations in vegetation or in surface soil. |